Measuring & Analyzing Greenhouse Gases: Behind the Scenes
Standards: The Secret of Success
We've used several different gas analyzers to measure the amount of a gas in our air sample. But these instruments only give us a measured quantity such as a voltage or the area under a curve in a chromatogram. How do we calculate the amount of the gas in units that really mean something? The answer is to use 'standards'.
The measurements we make are often called 'relative' measurements. That is, in order to get the actual amount of the gas, we need to compare that measurment relative to a measurement of a standard with known amounts of the same gas. For example, let's say we measured an unknown amount of CH4 and calculated the area under the CH4 peak to be 5,975,423 units. We next measure a known amount of CH4, say 1873.0 ppb, and calulated the area of the CH4 peak to be 6,053,834. We can calculate the mole fraction of the unknown by using the equation
Amount of CH4 = (5,975,423/6,053,834) * 1873.0 = 1848.74
By comparing our unknown measurement with a known amount, we can calculate the amount in our unknown sample. The actual number of standards used and the mathematical equation used varies depending on the instrument and the gas so that we get the most accurate value that we can. The value we end up with is known as a 'mole fraction' value.
Mole Fraction Units

The standards we use are tanks of air collected at Niwot Ridge in the Colorado Rockies that have been measured very accurately, so that the exact concentration of each gas in the tanks is known. Many different tanks have been created and precisely measured so that we have standards that cover the range of concentrations we encounter in the real world. To learn more about the creation and calibration of standards, read about the Calibration Laboratory.
Accuracy and Precision
We use infrared analyzers, fluorescence, gas chromatographs, and complicated measurement processes, so how do we know that after all of this we are getting the reliable answers? How do we know that these measurements are not affected by the small changes that the instruments experience over time (called drift), or by outright errors?
Our measurements need to be both accurate and precise. While the Greenhouse Gas Measurement System is very precise, unfortunately, it is not always very accurate on its own. That is, if we measure the same sample over and over again, we will always get very close to the same result, but that result may be slightly higher or lower than the true value. From day to day, the instruments may “drift” to higher or lower values. The good news is that we can solve this problem by comparing the measurements to standards that were measured using a different, very accurate manometric or gravimetric system. Between every sample measurement, we measure one of these standards. Both sample and standard measurements are very precise, and any bias (lack of accuracy) is the same for both the sample and standard. Using this, we can simply adjust, or “correct” the values of both sample and standard by the same amount, until the standard value is accurate. To be sure that we do this very well, we measure standards that have different concentrations of the gases so that our correction accounts for the possibility of instrument drift that may vary for different concentrations.
Of course, it is possible that our measurements and the correction to the standards is not as good as we would like. For example, there could be a noticeable error in the value of a standard reference gas. So in addition to that first set of standards that we use to make our instruments accurate, we have another set of standards, sometimes called “targets.” We measure air from these targets just like we measure real samples, and treat them as if we do not know what gas concentrations are in them. In reality, though, we have very precisely and accurately measured these targets in our calibration lab. The results from the targets tell us if our instruments are giving precise and accurate results. We continue to monitor the performance of the targets over time to tell us exactly how accurate and precise our results are, and whether there are any problems with the instruments.
Now, this seems like a reasonably reliable test, but the standard tanks and targets are filled differently than our samples, which are collected in flasks and twelve-pack. So we also fill flasks and twelve-packs with air from the standard tanks and measure them just like any other sample. This checks to make sure that the flasks and twelve-packs don’t change anything about our measurements.
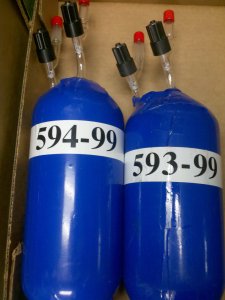
Just in case these first two tests are not enough, we also collect all our samples as pairs of flasks, filled at the same time at each field site.
Both flasks are measured, and we expect to get the same result. Any differences tell us about the precision of our entire system, from sample collection to measurement. Occasionally, large differences between the paired flasks are found, indicating a problem with the flask filling at the field site. At the tall tower sites, we collect samples in twelve-packs, and at the same time, we have continuous measurements of some of the gases on different instruments at the towers. The comparison between the two measurements gives yet another indication of how precise and accurate our measurements are.
Finally, at some of our sampling sites, we collect our flasks of air right next to samples collected by other greenhouse gas measurement labs around the world. Each lab measures its own samples, and then the results are compared to double-check that our flasks, measurement instruments, and standards are all in agreement.
All of these tests are put together to make sure that the measurements are as precise and accurate as possible. Now, when we measure our samples from around the world, we can be confident that the differences in greenhouse gas concentrations at different locations, and changes through time are reliable.
Next: The Calibration Laboratory