Barrow Radiation Climatology
This study was supported by the National Science Foundation under grant OPP-9321547 and NOAA's Global Monitoring Division.
It is well known that the polar regions play a critical role in the global energy balance by acting as a "cold sink", maintaining the north-south temperature gradients that drive Earth's oceanic and atmospheric circulations. Few long-term measurements of Arctic surface radiation balance components have been made, however, because of the region's remoteness and adverse conditions. Radiation measurements have been made at NOAA's Barrow Observatory (BRW) since June 1974. Analyses of these data provide an insight into the Arctic energy budget because perturbations in climate detected at BRW may reflect changes that may have occurred elsewhere in the high northern latitudes. Also, direct observations of surface radiative properties are necessary to provide "ground truth" for validating remotely sensed data, and to improve parameterizations used in climate models. The higher time resolution of surface data available from BRW enable important analyses that will lead to a better physical understanding of the unique climate feedback mechanisms that occur in polar regions.
Radiation and meteorological observations continue to be made at BRW in an effort to establish a long-term, comprehensive data set. Continuous monitoring will provide opportunities to detect climate change in this region of the Arctic.
A report (Stone et al., 1996) contains measured surface radiation budget (or balance) components with daily and monthly time resolution from BRW. See Figure 1 for an example. GMD's monitoring program and the data reduction and summary procedures are described. Tables and corresponding plots are presented showing annual cycles of several measured and derived radiation variables for three years, 1992, 1993, and 1994. In addition, coincident meteorological data are included, which are useful for interpreting the radiation measurements and how they vary on time scales of days to years.
The data presented in this report include the four basic components that constitute the net surface radiation balance; these being the upward and downward solar (or shortwave) and the upward and downward thermal infrared (or longwave) irradiances. In addition, measurements of direct-beam irradiance and surface albedo, derived from the ratio of the reflected solar radiation to the downwelling global irradiance, are presented. For descriptions of some of the instruments used, click here. Coincident meteorological information is given to provide a broader climatological context for evaluating the radiation balance at BRW. As such, this may be the most comprehensive report of its type for an Arctic site. Statistical summaries of the measured and derived surface variables deemed most important for the detection of climate change are presented in both tabular and graphical format.
Figure 1 (for 1994) shows a typical annual cycle of the radiation balance components, and also the total sky cover on a daily average time scale. Our measurements show a dramatic increase in net radiation each spring commencing at the end of May or first of June, coincident with maximum daily incident solar radiation (330-360 W m-2) at the surface. Snow melt follows soon after. Monthly mean net radiation does not peak until July each year (120-130 W m-2), which tends to be the least cloudy of summer months. Asymmetric to the peak in downward shortwave radiation, the downwelling thermal irradiance reaches a maximum, on average, during August (300-310 W m-2), which tends to be the cloudiest summer month. Year-to-year variations in the net radiation balance at BRW are found to be greatest during the winter months when the longwave components dominate. The day-to-day variability during winter is seen to correlate with transient events characterized by increased cloudiness, relatively warm temperatures, westerly winds, and a weakening of the surface-based temperature inversion. Positive cloud radiative forcing is evident during these events, and at times a slight positive net irradiance is observed at the surface (Stone, 1997).
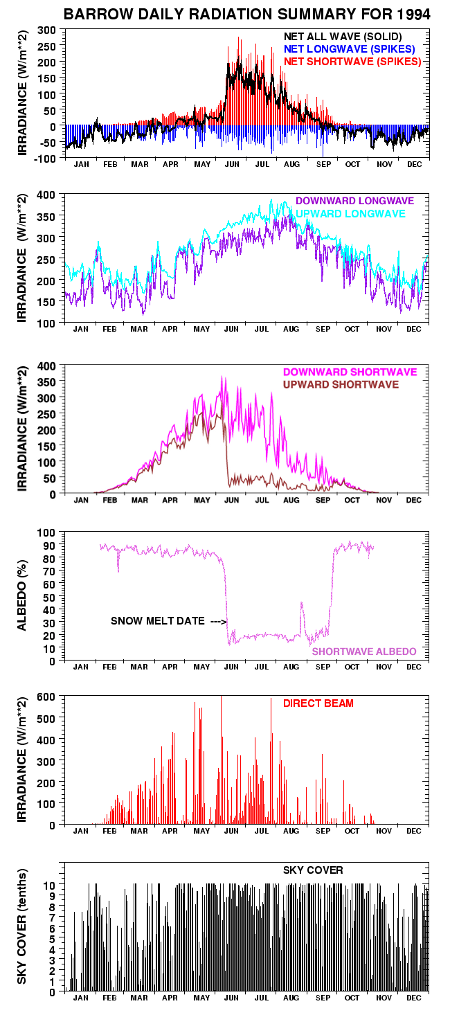
Panels from top to bottom are:
- Daily average net shortwave (red), net longwave (blue) and net radiation balance (black line)
- Daily average upward (cyan) and downward (violet) thermal infrared (or longwave) irradiances.
- Daily average upward (brown) and downward (magenta) solar (or shortwave) irradiances.
- Daily average surface albedo for BRW 1994, derived from the ratio of the reflected solar radiation to the downwelling global irradiance.
- Daily average direct beam irradiance.
- Daily average sky cover (cloudiness in tenths of total sky).
REFERENCES
Stone, R., T. Mefford, E. Dutton, D. Longenecker, B. Halter and D. Endres. Surface radiation and meteorological measurements: January 1992 to December 1994. NOAA Data Report ERL-CMDL-11, 81pp (1996).
Stone, R.S., Variations in western Arctic temperatures in response to cloud radiative and synoptic-scale influences, J. Geophys. Res., 102(D18),21,769-21,776, 1997.
RELATED LINKS:
ARM - North Slope of Alaska/Adjacent Arctic Ocean Site
RELATED ARTICLES:
- Albrecht, B., and S.K. Cox, 1976. Pyrgeometer data reduction and calibration procedures. Atmospheric Science Paper 251, Colorado State University, Fort Collins, CO, 48 pp.
- Dutton, E.G., 1993. An extended comparison between LOWTRAN7 computed and observed broadband thermal irradiances: Global extreme and intermediate surface conditions. J. Atmos. Oceanic Technol., 10(3), 326-336.
- Dutton, E.G., and D.J. Endres, 1991. Date of snowmelt at Barrow, Alaska, U.S.A. Arc. Alp. Res., 23(1), 115-119.
- Dutton, E.G., R.S. Stone, and J.J. DeLuisi, 1989. South Pole surface radiation balance measurements, April 1986 to February 1988. NOAA Data Rep. ERL ARL-17, NOAA/ERL, Air Resources Laboratory, Silver Spring, Md, 49 pp.
- Dutton, E.G., J.J. DeLuisi, and D.J. Endres, 1985. Solar radiation at the Barrow, AK, GMCC baseline observatory 1976-1978. NOAA Data Rep. ERL ARL-6, NOAA/ERL Air Resources Laboratory, Boulder, CO, 112 pp.
- Halter, B.C., and J.M. Harris, 1983. On the variability of atmospheric carbon dioxide concentration at Barrow, Alaska during winter. J. Geophys. Res., 88, 6858-6864.
- Halter, B.C., and J.T. Peterson, 1981. On the variability of atmospheric carbon dioxide concentration at Barrow, Alaska during winter. Atmos. Environ., 15, 1391-1399.
- Harris, J.M., and J.D.W. Kahl, 1994. Analysis of 10-day isentropic flow patterns for Barrow, Alaska: 1985-1992. J. Geophys. Res., 99(D12), 25,845-25,855.
- Herbert, G.A., E.R. Green, G.L. Koenig, and K.W. Thaut, 1986. Monitoring instrumentation for the continuous measurement and quality assurance of meteorological observations. NOAA Tech. Memo. ERL ARL-148, NOAA/ERL Air Resources Laboratory, Boulder, CO, 44 pp.
- Johannessen, O.M., M. Miles, and E. Bjorgo, 1995. The Arctic's shrinking sea ice. Nature, 376, 126-127.
- Kahl, J.D., 1990. Characteristics of the low-level temperature inversion along the Alaskan Arctic coast. Int. J. Climatol., 10, 537-548.
- Kelly, J.J., D.T. Baily, and B.J. Lieske, 1964. Radiative energy exchange over Arctic land and sea. Data 1962 Sci. Rep., Dept. Atmos. Sci., Univ. of Washington, Seattle, 205 pp.
- Key, J.R., A.J. Schweiger and R.S. Stone, 1997. Expected uncertainty in satellite-derived estimates of the surface radiation budget at high latitudes. J. Geophys. Res., 102(C7), 15,837-15,847.
- Key J.R., R.A. Silcox, and R.S. Stone, 1996. Evaluation of surface radiative flux parameterizations for use in sea ice models. J. Geophys. Res. (in press).
- Klein, W.H., and B. Goldberg, 1978. Solar radiation measurements, 1968-1977. Reports, Smithson. Rad. Bio. Lab., Rockville, MD.
- Maslanik, J.A., M.C. Serreze, and R.G. Barry, 1996. Recent decreases in summer Arctic ice cover and linkages to anomalies in atmospheric circulation. Geophys. Res. Lett. (submitted).
- Maykut, G.A., and P.E. Church, 1973. Radiation climate of Barrow, Alaska, 1962-66. J. Appl. Meteorol., 12, 620-628.
- Nelson, D., 1996. NOAA Solar Radiation Calibration Facility, 1985-1996. Unpublished manuscript, NOAA/ERL Climate Monitoring and Diagnostics Laboratory, Boulder, CO.
- Peterson, J.T., and R.M. Rosson, 1993. Climate Monitoring and Diagnostics Laboratory No. 21, Summary Report 1992. NOAA/ERL, Boulder, CO, 131 pp.
- Peterson, J.T., and R.M. Rosson, 1994. Climate Monitoring and Diagnostics Laboratory No. 22, Summary Report 1993. NOAA/ERL, Boulder, CO, 152 pp.
- Rossow, W.B., 1992. Polar cloudiness: Some results from ISCCP and other cloud climatologies. Preprints, Third Conf. on Polar Meteorology and Oceanography, 29 Sept-2 Oct. 1992, Portland, Oregon. American Meteorology Society, Boston, 218 pp.
- Serreze, M.C., J.A. Maslanik, J.R. Key, R.F. Kokaly, and D.A. Robinson, 1995. Diagnosis of record minimum in Arctic sea ice area during 1990 and associated snow cover extremes. Geophys. Res. Lett., 22(16), 2183-2186.
- Stokes, G.M., and S.E. Schwartz, 1994. The Atmospheric Radiation Measurement (ARM) Program: Programmatic background and design of the cloud and radiation test bed. Bull.
- Thornthwaite, C., and J. Mather, 1958. Microclimatic investigations at Point Barrow, Alaska, 1957- Amer. Meteorol. Soc., 75, 1201-1221.
- 1958. Publications in Climatology, 11(2), Drexel Institute of Technology, Laboratory of Climatology, Centerton, NJ, 237 pp.
- Weller, G., and B. Holmgren, 1974. The microclimates of the Arctic tundra. J. Appl. Meteorol., 13, 854-862.
- Wendler, G., F.D. Eaton, and T. Ohtake, 1981. Multiple reflection effects on irradiance in the presence of arctic stratus clouds. J. Geophys. Res., 83, 2049-2057.
- Wielicki, B.A., R.D. Cess, M.D. King, D.A. Randall, and E.F. Harrison, 1995. Mission to Planet Earth: Role of clouds and radiation in climate. Bull. Amer. Meteorol. Soc., 76 (11), 2125-2153.