Research Themes
Guiding Recovery of Stratospheric Ozone
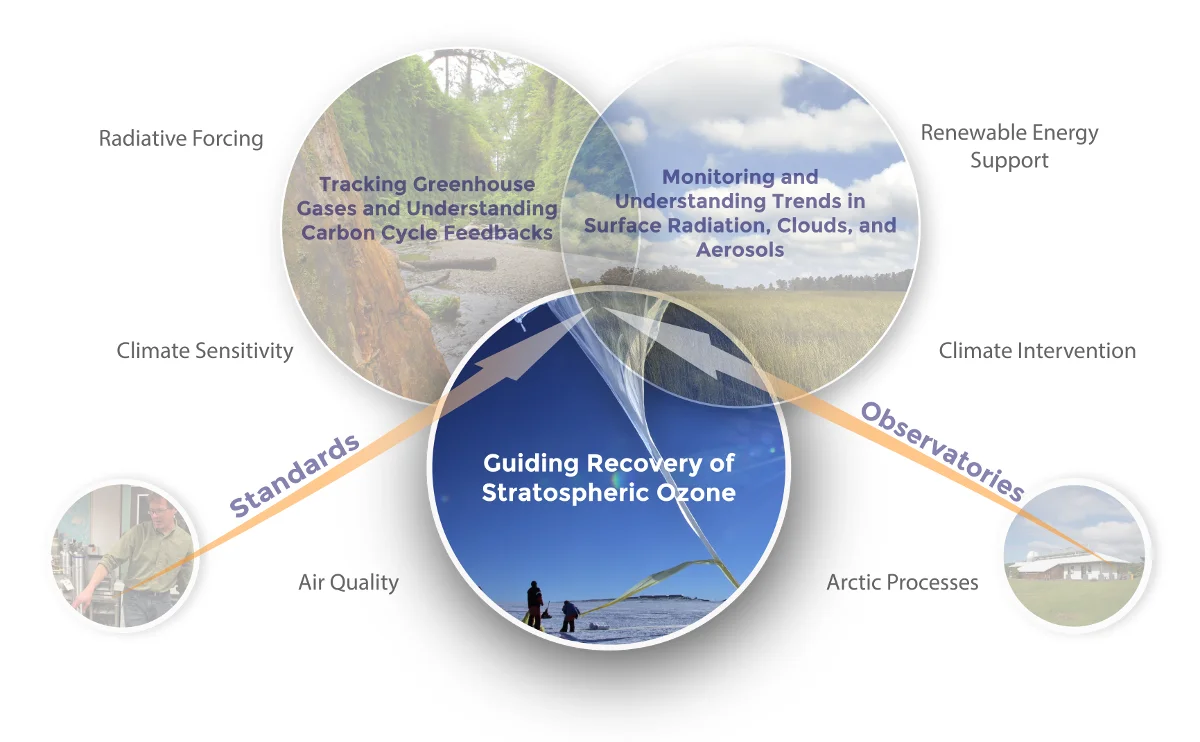
Depletion of stratospheric ozone can result in enhanced UV radiation levels that increase skin cancer rates and adversely affect organisms and ecosystems. Concern over these effects provided impetus for ratifying the 1987 Montreal Protocol, enacting the U.S. Clean Air Act of 1990, and initiating GML’s global-scale monitoring of stratospheric ozone and the gases responsible for its destruction.
GML has developed a carefully designed network to monitor variations in ozone, ozone-depleting substances, stratospheric aerosols, and UV radiation. GML research has been critical in determining longterm changes in concentrations of stratospheric ozone and chemicals causing ozone depletion. Our unique long-term observational records have led to an improved understanding of the production and fate of stratospheric ozone and the compounds and processes that influence ozone’s abundance. These advances have furthered our understanding of the fundamental atmospheric processes affecting stratospheric ozone and provide usable information to policy makers for guiding the recovery of the ozone layer.
GML conducts year round balloon-borne vertical structure and total column optical measurements of ozone over the South Pole. During the winter (preceding the early springtime Antarctic ”ozone hole”), satellites are unable to measure polar ozone without sunlight. GML monitors stratospheric ozone at lower latitudes and in the Arctic, measures the gases responsible for depletion of stratospheric ozone, and monitors changes in ultraviolet radiation that is controlled by the amount of ozone in the stratosphere. As such, understanding the production and fate of ozone and the ozone-depleting compounds is a focal point of GML research.
Ground based measurements of total-column ozone have been made for over 50 years with the Dobson spectrophotometer; the 14-station GML Cooperative Dobson Network is a significant portion of the global Dobson network as are the six GML balloon-borne ozonesonde stations. These stratospheric ozone measurements are linked to the world calibration standards maintained by GML as are a preponderance of the stations in other international global networks.
Three gases that make a significant contribution to stratospheric ozone depletion, CFC-11, CFC-12 and N2O, have been monitored by GML since the mid-1970s. Since then, numerous additional CFCs, HCFCs, and other halogenated gases have been incorporated into the measurement program as the number of monitoring sites increased. Most of the gases that are responsible for depleting stratospheric ozone are anthropogenic, but some, such as methyl bromide and methyl chloride, have natural contributions as well.
Is the fully revised and amended Montreal Protocol successfully reducing the threat to stratospheric ozone posed by ozone-depleting substances?
Relevance: The intention of international and national controls on the production of ozone-depleting substances (ODSs) is to reduce atmospheric concentrations of these substances and allow for the stratospheric ozone layer to recover. This will bring UV radiation and, potentially, skin cancer rates back to their pre-ozone hole levels, and reduce the risk to agriculture and ecosystems.
Actions Taken: GML continues to monitor the abundance and global distribution of ODSs at surface sites across the globe to ascertain if global production controls are having their desired effect. Changes in the summed atmospheric concentration of ODSs are tracked and updated annually with GML’s Ozone-Depleting Gas Index (ODGI). GML also coordinates with other groups (e.g., the Advanced Global Atmospheric Gases Experiment (AGAGE)) to compare independent global measurements and calibration scales for ODSs.
What we’ve discovered: GML results have demonstrated the ongoing success of the Montreal Protocol and U.S. Clean Air Act in reducing the concentrations of ODSs and, therefore, the threat they pose to the ozone layer. The summed concentration of ODSs has decreased steadily for over two decades, although increases in some short-lived chlorinated gases not controlled under the Protocol have been observed.
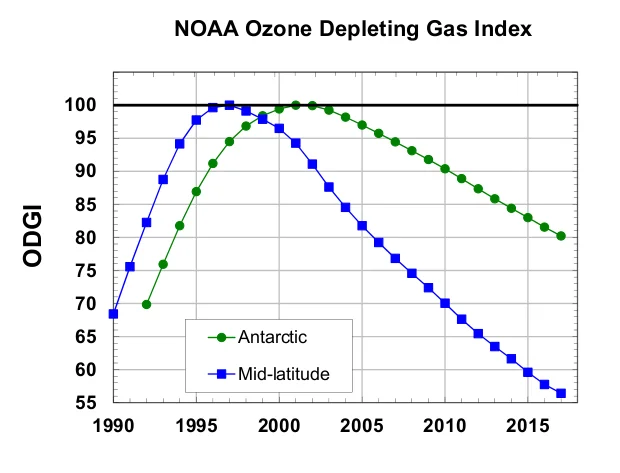
Is stratospheric ozone recovering as expected?
Relevance: The implementation of the Montreal protocol and its amendments bans ozone-depleting substances from production. Levels of these harmful substances are decreasing (Q1), but still remain relatively high in the stratosphere. These reductions are expected to increase stratospheric ozone levels by the middle of this century and bring UV radiation and skin cancer rates back to their pre-ozone hole levels. However, changes in the atmospheric greenhouse gases are shown to impact on recovery of stratospheric ozone. The variability in future ODS and GHG levels in the atmosphere makes it difficult to predict ozone recovery and thus requires ozone levels to be continuously monitored. The Ozone-Depleting Gas Index (ODGI) vs. time calculated for the Antarctic and mid-latitude stratosphere. The ODGI is derived directly from the Equivalent Effective Stratospheric Chlorine (EESC) determined from GML’s atmospheric surface observations.
Actions Taken: GML’s global, long-term monitoring of ozone is a core element of international ozone measurement networks. The results from GML’s program help distinguish between short and longterm processes, and enable the proper attribution of ozone changes to chemical and climate-related processes. GML monitors decadal change in ozone at several research sites at strategic locations around the globe. Stratospheric and total column ozone trends are assessed from long-term, calibrated and quality assured records of ozonesonde balloon profiles, ground-based total column and vertical profile (Dobson Umkehr) ozone measurements. GML provides the only year-round measurements at the South Pole to track ozone hole recovery.
What we’ve discovered: Historically, ozone decreased substantially as the concentrations of ODSs increased. After ODS concentrations peaked and began to decrease in the early 2000s, GML-measured ozone levels in Antarctica stopped decreasing and have since shown the first signs of recovery. More recently, there is mounting evidence that ozone is recovering in the stratosphere. Moreover, at the South Pole station, GML ozonesonde records collected during September show statistically significant ozone recovery since 2000. Nonetheless, large year-to-year variability in the stability of the Polar vortex due to climatically changing meteorological conditions and associated variability in ozone depletion levels requires continuous monitoring of stratospheric ozone.
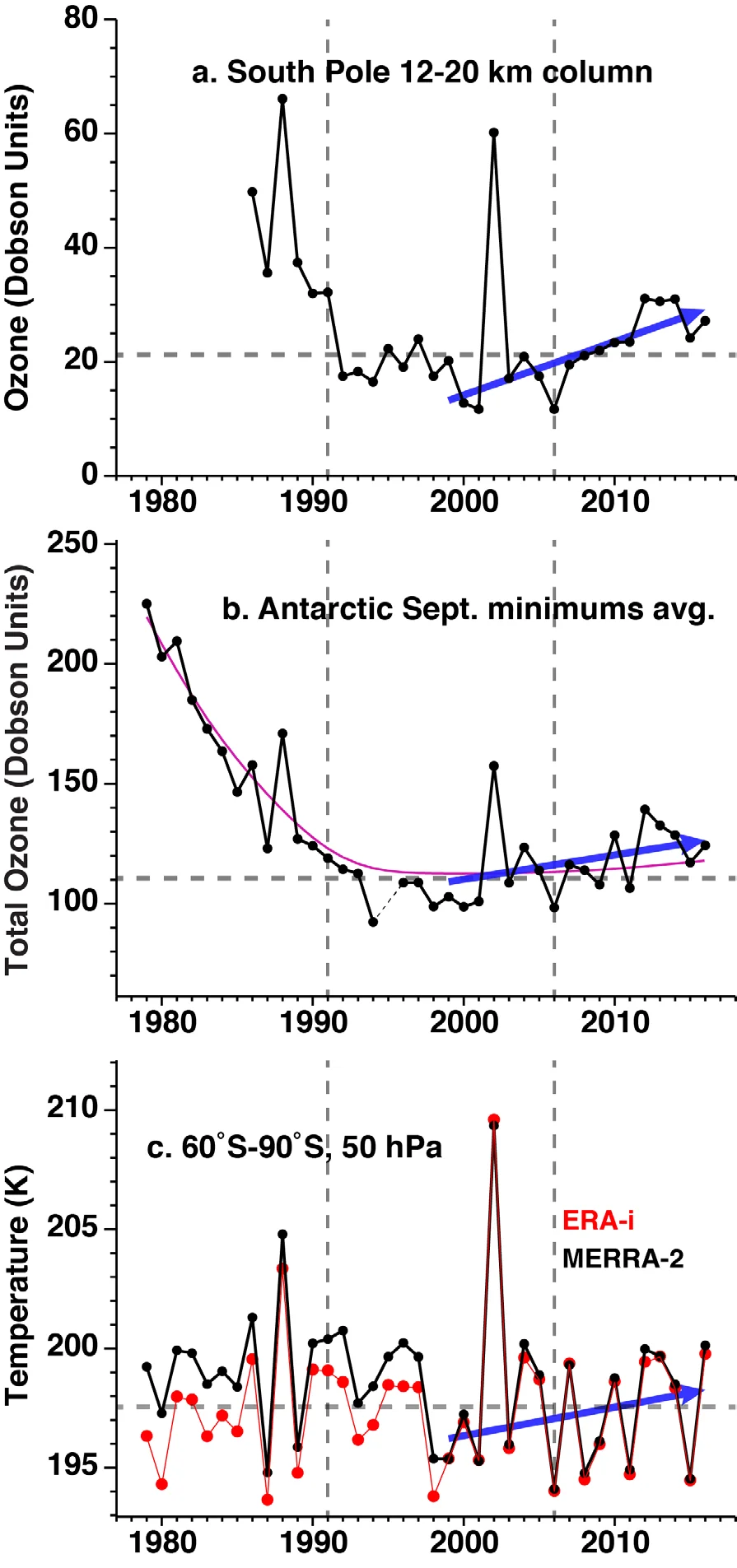
How is the Brewer-Dobson circulation changing in response to increased greenhouse gas concentrations and how has this altered the global distribution of ozone and the atmospheric lifetimes of ozone-depleting and climate altering gases?
Relevance: Mean Brewer-Dobson circulation (BDC) affects ozone recovery in the stratosphere by modifying the lifetimes of the ozone-depleting species, by changing the local, highly nonlinear ozone chemistry, and by directly changing the distribution of ozone-depleting gases brought up from the troposphere. Tropical lower stratospheric water vapor is a sensitive indicator of changes in the strength of the BDC that alter cold point temperatures in the tropics. A larger impact of the change in BDC is that it affects circulation in the troposphere. The BDC appears to be increasing at altitudes below 25 km based on estimates of the mean age of stratospheric air. This result is expected from Global Climate Models as the lower tropospheric temperature increases from increased emissions of GHGs. In contrast, recovery of stratospheric ozone could weaken the BDC.
Actions Taken: GML observations, along with others, have been used to infer changes in the mean-age of stratospheric air. However, stratospheric observations of trace gases that would help identify trends in BDC are not performed on a regular basis. GML developed a plan to determine BDC changes using stratospheric age of air using AirCores and is currently working to support a three-year experimental campaign to measure numerous trace gases using AirCores and balloon-borne instruments at 4-5 locations around the world.
What we’ve discovered: Ozone depletion and climate models need to realistically take into account stratospheric circulation and its changes. At present, stratospheric circulation is poorly defined by stratospheric wind data assimilated into 3D models. The mean age of stratosphere air, derived from measurements of trace gases, shows that stratospheric circulation has been changing over the last 37 years. We have observed a decrease in the mean age of air at altitudes <25 km.
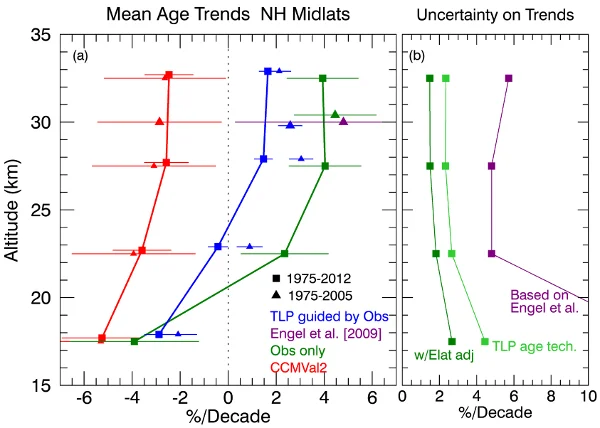
How sensitive is the oxidative capacity of the atmosphere and how is it changing over time?
Relevance: Atmospheric oxidation processes constantly cleanse the atmosphere of thousands of tons of reactive gases emitted by humans each year, including some that impact on stratospheric ozone. These human-derived pollutant emissions in turn affect the oxidative capacity of the atmosphere and its ability to cleanse itself of these pollutants.
Actions Taken: To track the oxidative capacity of the atmosphere, GML has invested in long-term, high-quality measurements of short-lived halogenated gases (e.g., methyl chloroform), HCFCs, methane, surface ozone, and tropospheric and stratospheric ozone far away from the pollution sources and in locations with background conditions. The changes in atmospheric composition are regularly evaluated. The International Global Atmospheric Chemistry (IGAC) TOAR (Tropospheric Ozone Assessment Report, 2017) used GML long-term records to evaluate changes in the global and regional tropospheric ozone burden.
What we’ve discovered: Estimates based on global measurements of methyl chloroform have led to the conclusion that the oxidizing capacity of the atmosphere is well buffered against large interannual changes. Long-term changes, specifically of hydroxyl radical concentrations, are still possible. Ultimately, the stability of this oxidizing capacity will depend on the potential for significant increases in pollutant emissions and the resulting build up in background tropospheric ozone, as developing countries continue to industrialize. GML records show that the tropospheric ozone burden is changing regionally and globally, while the location of the enhanced ozone precursors has recently moved to the tropics from mid-latitudes, where they have a larger impact on the global tropospheric ozone burden. Changes in the upper and lower troposphere have also been observed with satellites, but those records are not mature and differ from one another. GML’s long-term and globally distributed tropospheric ozone measurements provide critical information essential for improvement of climate chemistry and chemical transport models’ prediction skill.
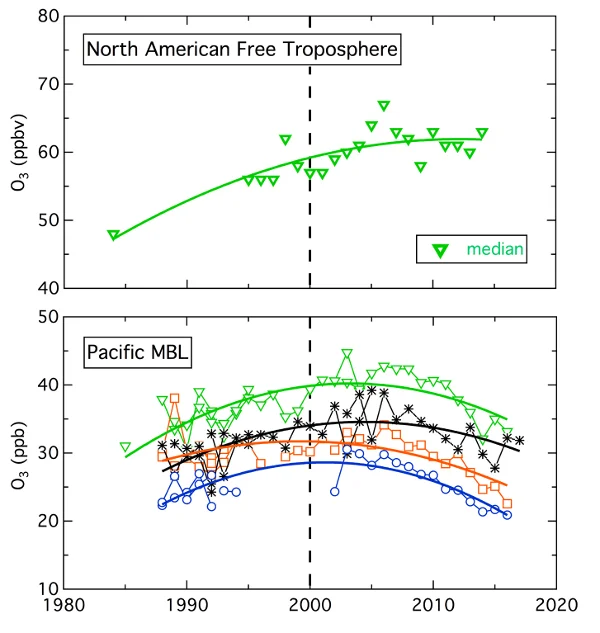